Research directions
The development of the IPET (Individual Particle Electron Tomography) technique aims to determine the high-resolution structure of a single molecule without the need for particle averaging:
Macromolecules play a critical role in cellular activity through their unique 3D structures and conformational changes. However, traditional techniques like X-ray crystallography, NMR, and single-particle electron microscopy have limitations in determining the structures of flexible and dynamic macromolecules due to their large-scale structural heterogeneity. To address this limit, the IPET technique was developed by the lab (Zhang and Ren, PLoS One, 2012). It allows to characterize the structure and variety at an intermediate resolution (1-3 nm) without pre-existing models or particle averaging. Through IPET, the lab has revealed the structural dynamics of flexible macromolecules such as antibodies (Zhang, et al.,Sci. Rep., 2015; Zhang, et al., Ana. Chem., 2017; Lei, et al., Sci. Rep., 2019); Tong, et al., Sci. Rep., 2013, D/RNA nanostructures (Zhang, et al., Nat. Comm., 2016; Lei, et al., Nat. Comm., 2018; Wang, et al., Nat. Comm., 2021; McRae, et .al., Nat. Nano., 2023; Liu, et .al., Nat. Comm., 2024), Nucleosome arrays (Zhang, et al.,Mol. Cell, 2022; Zhang, et al.,Nat. Comm., 2024); lipoproteins (Yu, et al.,JLR, 2016; Lei, et al.,BBA lipids, 2019; Wu, et al.,Sci. Rep., 2018; Zhang, et al.,Sci. Rep., 2014), flexible proteins (Lee, et al.,JBC, 2023; (Liu, et al.,JBM, 2018; Lu, et al.,JBC, 2016; Lu, et al.,JBC, 2014; Zhang, et al.,BBA lipids, 2018), and nanoparticles (Xu, et al.,Nat. Energy, 2023).
Liquid-TEM imaging the real-time dynamic structure of the liquid-vapor interface Due to the high vacuum of the TEM column, liquid samples can quickly evaporate before imaging, making TEM imaging of both materials and biological samples in liquid-phase challenging. To challenge this difficulty, we developed a simple liquid cell to encapsulate the liquid-phase sample and examine it by TEM. This method allows us to observe the real-time fluctuation and dynamic structure of the liquid-vapor interface of molten sodium at nanometer resolution (Ren, et al., Sci. Rep., 2020). The method has also been used to examine biological samples, including proteins, viruses, bacteria, and cells in the liquid-phase under room or physiological temperature.
Designing CETP inhibition is a potential approach for treating cardiovascular diseases. Cholesteryl Ester Transfer Protein (CETP) is a major contributor to cardiovascular disease (CVD) and familial hyperlipidemia by facilitating the transfer of cholesterol between lipoproteins. Despite the failure of five CETP inhibitors in large clinical trials, our research has shown that CETP penetrates into HDL and LDL/VLDL, creating a tunnel for cholesterol ester transfer between lipoproteins (Zhang, et al., Nat. Chem. Biol., 2012). Through our studies on CETP inhibition mechanisms (Zhang, et al., BBA Lipids, 2017) and similar protein, phospholipid transfer protein (PLTL) (Zhang, et al. BBA Lipids, 2018), we have discovered potential antibody inhibitors as regulators of plasma lipid levels for the treatment of CVD. Our research has also revealed that CETP binds to HDL through hydrophobic interactions rather than protein-protein interactions, and that several key residues play a vital role in cholesterol ester transfer, as revealed by molecular dynamics (MD) simulations (Zhang, et al. Sci. Rep., 2015; Lei, et al. JBC, 2016).
Theory of everything: the principle of least action on multiple dimensional space The pursuit of a comprehensive theory that can unify and explain all physical phenomena in the universe has been a longstanding goal of physicists. General Relativity and Quantum Mechanics have emerged as the two theoretical frameworks that come closest to achieving this ultimate theory. One possible approach to finding such a theory is to seek a connection between the fundamental equations in physics. In 2017, Ren and Duan demonstrated that the extended harmonic mapping (EHM) method, which uses harmonic maps with potential, could link together the equations of General Relativity, chaos, and Quantum Mechanics through a universal geodesic equation (Ren and Duan, Chaos, Solitons & Fractals, 2017, 103: 567–570). This equation, expressed as Euler-Lagrange equations on a Riemannian manifold, was derived from the principle of least action. In 2020, Ren et al. demonstrated that over ten fundamental equations from various branches of physics, including classical mechanics, fluid physics, statistical physics, astrophysics, quantum physics, and General Relativity, could be connected through the same universal geodesic equation (Zhai, et. al., Sci. Rep., 2020, 10, 18281). This connection outlines a family tree of physics equations and reveals an alternative underlying rule of the universe: the principle of least action on a Finsler manifold.
The development of the IPET (Individual Particle Electron Tomography) technique aims to determine the high-resolution structure of a single molecule without the need for particle averaging:
Macromolecules play a critical role in cellular activity through their unique 3D structures and conformational changes. However, traditional techniques like X-ray crystallography, NMR, and single-particle electron microscopy have limitations in determining the structures of flexible and dynamic macromolecules due to their large-scale structural heterogeneity. To address this limit, the IPET technique was developed by the lab (Zhang and Ren, PLoS One, 2012). It allows to characterize the structure and variety at an intermediate resolution (1-3 nm) without pre-existing models or particle averaging. Through IPET, the lab has revealed the structural dynamics of flexible macromolecules such as antibodies (Zhang, et al.,Sci. Rep., 2015; Zhang, et al., Ana. Chem., 2017; Lei, et al., Sci. Rep., 2019); Tong, et al., Sci. Rep., 2013, D/RNA nanostructures (Zhang, et al., Nat. Comm., 2016; Lei, et al., Nat. Comm., 2018; Wang, et al., Nat. Comm., 2021; McRae, et .al., Nat. Nano., 2023; Liu, et .al., Nat. Comm., 2024), Nucleosome arrays (Zhang, et al.,Mol. Cell, 2022; Zhang, et al.,Nat. Comm., 2024); lipoproteins (Yu, et al.,JLR, 2016; Lei, et al.,BBA lipids, 2019; Wu, et al.,Sci. Rep., 2018; Zhang, et al.,Sci. Rep., 2014), flexible proteins (Lee, et al.,JBC, 2023; (Liu, et al.,JBM, 2018; Lu, et al.,JBC, 2016; Lu, et al.,JBC, 2014; Zhang, et al.,BBA lipids, 2018), and nanoparticles (Xu, et al.,Nat. Energy, 2023).
Liquid-TEM imaging the real-time dynamic structure of the liquid-vapor interface Due to the high vacuum of the TEM column, liquid samples can quickly evaporate before imaging, making TEM imaging of both materials and biological samples in liquid-phase challenging. To challenge this difficulty, we developed a simple liquid cell to encapsulate the liquid-phase sample and examine it by TEM. This method allows us to observe the real-time fluctuation and dynamic structure of the liquid-vapor interface of molten sodium at nanometer resolution (Ren, et al., Sci. Rep., 2020). The method has also been used to examine biological samples, including proteins, viruses, bacteria, and cells in the liquid-phase under room or physiological temperature.
Designing CETP inhibition is a potential approach for treating cardiovascular diseases. Cholesteryl Ester Transfer Protein (CETP) is a major contributor to cardiovascular disease (CVD) and familial hyperlipidemia by facilitating the transfer of cholesterol between lipoproteins. Despite the failure of five CETP inhibitors in large clinical trials, our research has shown that CETP penetrates into HDL and LDL/VLDL, creating a tunnel for cholesterol ester transfer between lipoproteins (Zhang, et al., Nat. Chem. Biol., 2012). Through our studies on CETP inhibition mechanisms (Zhang, et al., BBA Lipids, 2017) and similar protein, phospholipid transfer protein (PLTL) (Zhang, et al. BBA Lipids, 2018), we have discovered potential antibody inhibitors as regulators of plasma lipid levels for the treatment of CVD. Our research has also revealed that CETP binds to HDL through hydrophobic interactions rather than protein-protein interactions, and that several key residues play a vital role in cholesterol ester transfer, as revealed by molecular dynamics (MD) simulations (Zhang, et al. Sci. Rep., 2015; Lei, et al. JBC, 2016).
Theory of everything: the principle of least action on multiple dimensional space The pursuit of a comprehensive theory that can unify and explain all physical phenomena in the universe has been a longstanding goal of physicists. General Relativity and Quantum Mechanics have emerged as the two theoretical frameworks that come closest to achieving this ultimate theory. One possible approach to finding such a theory is to seek a connection between the fundamental equations in physics. In 2017, Ren and Duan demonstrated that the extended harmonic mapping (EHM) method, which uses harmonic maps with potential, could link together the equations of General Relativity, chaos, and Quantum Mechanics through a universal geodesic equation (Ren and Duan, Chaos, Solitons & Fractals, 2017, 103: 567–570). This equation, expressed as Euler-Lagrange equations on a Riemannian manifold, was derived from the principle of least action. In 2020, Ren et al. demonstrated that over ten fundamental equations from various branches of physics, including classical mechanics, fluid physics, statistical physics, astrophysics, quantum physics, and General Relativity, could be connected through the same universal geodesic equation (Zhai, et. al., Sci. Rep., 2020, 10, 18281). This connection outlines a family tree of physics equations and reveals an alternative underlying rule of the universe: the principle of least action on a Finsler manifold.
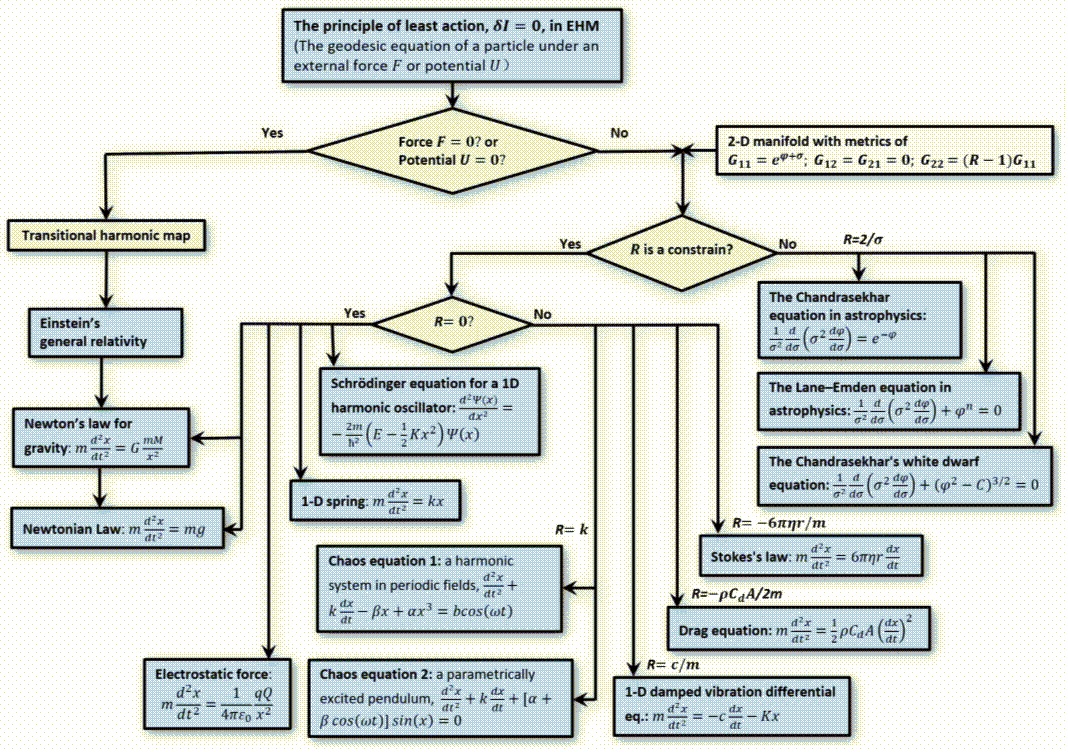