Single-molecule 3D structure determination by individual-particle cryo-electron tomography (IPET)
Macromolecules, such as proteins and nucleic acids, play a critical role in cellular activity through their unique 3D structures and conformational changes. Understanding their functions requires the characterization of their structure and dynamics. While current techniques, including X-ray crystallography and cryo-electron microscopy (cryo-EM), can determine high-resolution structures of rigid-body molecules, revealing the low-resolution structures of flexible macromolecules with continuously changing conformations, such as lipoproteins, antibodies, neuronal proteins, and DNA/RNA nanostructures, remains challenging. This is because the current determination methods rely on an averaging process, where thousands of homogeneous particles must be selected from a large pool of heterogeneity for averaging. Although averaging can improve the signal-to-noise ratio to achieve high-resolution structures of rigid-body portions, it can also result in missing density of flexible domains, anisotropic resolution distribution, and failure to determine structures with high-energy states (low population).
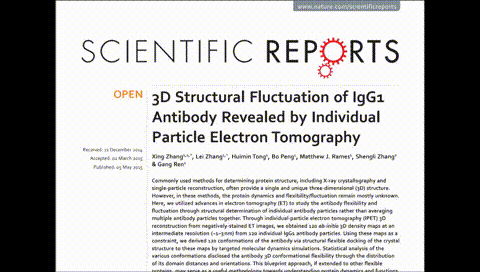
The objective of this laboratory is to develop a cryo-electron tomography (cryo-ET) method to determine the 3D structure of a single molecule, named the individual-particle electron tomography (IPET) method (Zhang, et al., PLoS One, 2012), without averaging. Structure determination of a single molecule plays an important role in understanding macromolecular dynamics and the function/mechanism during chemical reactions. However, this determination is not trivial, but rather challenging due to the extremely low signal-to-noise ratio (SNR) of a single molecule caused by the electron dose limit necessary for preventing radiation damage. Over the past decade, this laboratory has reported a series of TEM techniques to improve the TEM capability to image a single molecule.
The reported TEM techniques include: i) the development of focused 3D reconstruction algorithms to reduce the large-scale image distortion/diffraction effect during 3D reconstruction (Zhang, et al., PLoS One, 2012); ii) a fully mechanical-controlled TEM software for cryo-ET data acquisition to prevent beam-hysteresis caused by beam-coherence reduction during tilting (Liu, et al., Sci Rep., 2016); iii) a noise-reducing algorithm to enhance the signal-to-noise ratio (SNR) of a single molecule (Wu, et al., Sci Rep., 2018); iv) a missing-wedge correction method to reduce the enlargement artifact induced by the tilt limit (Zhai, et al., Sci Rep., 2020); v) a cryo-positive staining (cryo-PS) method for imaging the detailed structure of a single-instant small protein (Zhang, et al., Nat Chem Bio., 2012); vi) an optimized negative-staining (OpNS) method to reduce the relaxation artifact of lipoproteins (Rames, et al., J Vis Exp., 2014; Zhang, et al., J Lipid Res., 2010); vii) a simple liquid-cell TEM method for real-time imaging the dynamics of liquid-vapor interface (Ren, et al., Sci Rep., 2020).
Based on current improvements, this laboratory has characterized the structural variety of several flexible macromolecules, including antibodies (Zhang, et al., Sci Rep., 2015; Tong, et al., Sci Rep., 2013; Zhang, et al. Anal Chem., 2017; Lei, at al., Sci Rep., 2020), D/RNA-related macromolecules (Zhang, et al., Nat comm., 2016; Lei, et al., Nat comm., 2021; Wang, et al., Nat comm., 2022; Zhang, et al., Mol Cell., 2022; McRae, et al., Nat Nano., 2023), lipoproteins (Zhang, et al., Sci Rep., 2015; Yu, et al.,J Lipid Res., 2016; Lei, et al.,BBA lipids, 2019), and neuron proteins (Lu, et al., J Bio Chem., 2014; Lu, et al., J Biol Chem., 2016; Lee, et al., J Biol Chem., 2023).
The development of IPET capability for high-resolution structure determination of a single protein is crucial in translating the current static structures into future dynamic structures, which is essential for understanding the processes of synthesis, folding, and chemical reactions of macromolecules.